Unseen but Dangerous: Understanding the Health Risks of Ultrafine Particles in Ambient Air
- Expert Article
Discover how these tiny pollutants penetrate our bodies, impacting respiratory and cardiovascular health, and learn about the cutting-edge technologies that can help us monitor and mitigate their effects.
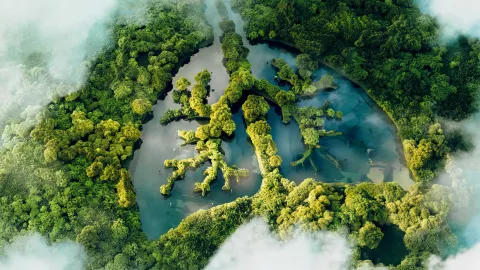
Ultrafine particles (UFPs), defined as particles less than 0.1 microns in size, are a pervasive component of the ambient air, stemming from both natural processes and human activities such as transportation, industrial emissions, and cooking. Despite their microscopic size, UFPs pose significant health risks that necessitate focused monitoring and mitigation efforts. This article explores the nature of UFPs, their health impacts, the biological mechanisms involved, and the importance of advanced monitoring technologies.
What Are Ultrafine Particles (UFPs)?
UFPs represent the smallest fraction of particulate matter (PM) in the air. Their tiny size allows them to behave differently from larger particles such as PM10 and PM2.5. Traditional PM2.5 measurements often fail to capture the true concentration and impact of UFPs due to their extremely low mass (Hinds, 1999). As such, even in environments where PM2.5 levels appear low, UFPs can still be present in high numbers, potentially leading to significant health risks.
Sources of Ultrafine Particles
UFPs originate from various sources, both anthropogenic and natural. Major human activities contributing to UFP emissions include:
- Transportation: Vehicle exhaust is a significant source of UFPs, especially from diesel engines (Kittelson, 1998).
- Industrial Processes: Factories and power plants emit UFPs during combustion and other processes (Sioutas et al., 2005).
- Cooking: Both indoor and outdoor cooking activities can release substantial amounts of UFPs (Buonanno et al., 2009).
- Natural sources of UFPs include volcanic eruptions, wildfires, and sea spray, among others (Seinfeld & Pandis, 2016)
Recent research has demonstrated that UFPs can penetrate deeply into the respiratory system and translocate to other organs, including the brain. These particles are associated with a range of adverse health effects, including cardiovascular, respiratory, and neurological diseases (Oberdörster et al., 2005).
Penetration and Translocation
Due to their small size, UFPs can easily bypass the body's natural defense mechanisms. They can penetrate deep into the alveolar region of the lungs and enter the bloodstream, subsequently reaching various organs. This translocation capability increases their potential to cause widespread damage (Geiser & Kreyling, 2010).
Oxidative Stress and Inflammation
UFPs generate reactive oxygen species (ROS) upon inhalation, leading to oxidative stress and inflammation. Oxidative stress occurs when there is an imbalance between ROS production and the body's ability to detoxify these reactive intermediates or repair the resulting damage. This process can result in cellular and tissue damage, contributing to diseases such as atherosclerosis, hypertension, and even cancer (Li et al., 2003).
Epigenetic Changes
Exposure to UFPs can lead to epigenetic modifications, which are changes in gene expression without altering the DNA sequence. These changes can disrupt normal cell function and lead to chronic diseases. For instance, studies have shown that UFP exposure can alter the methylation patterns of genes involved in inflammation and cancer (Vezzani et al., 2022).
Respiratory and Cardiovascular Diseases
Numerous studies have linked UFP exposure to respiratory illnesses such as asthma, chronic bronchitis, and pneumonia. UFPs can aggravate existing respiratory conditions and increase susceptibility to infections. Additionally, cardiovascular diseases such as congestive heart failure and coronary artery disease have been associated with UFP exposure due to their ability to induce oxidative stress and systemic inflammation. For example, Hong et al. (2020) found that ultrafine particles can cause significant damage to the respiratory system and are associated with increased risk of bronchial asthma, chronic obstructive pulmonary disease (COPD), and lung cancer. These particles penetrate deep into the lungs, leading to systemic inflammation and exacerbating pre-existing respiratory conditions.
Furthermore, UFPs can also affect cardiovascular health by inducing oxidative stress and inflammation. Studies have shown that these particles can enter the bloodstream, causing vascular inflammation and contributing to conditions such as atherosclerosis and heart disease. In a study by Ostro et al. (2015), long-term exposure to ultrafine particles was linked to increased incidences of respiratory diseases and cardiovascular conditions, highlighting the critical need for monitoring and mitigating UFP pollution (Hong et al., 2020; Weichenthal et al., 2017).
Neurological Effects
One of the most concerning aspects of UFPs is their ability to cross the blood-brain barrier, a protective shield that prevents most substances in the blood from entering the brain. Studies have found that UFPs can reach the brain and potentially cause neuroinflammation, which is linked to conditions such as Alzheimer's disease and other neurodegenerative disorders (Calderón-Garcidueñas et al., 2008).
Given the unique health risks posed by UFPs, it is crucial to include UFP measurements in regular ambient air quality monitoring. Accurate and real-time data on UFP concentrations can help identify high-risk areas and implement necessary interventions to protect public health.
Monitoring Benefits
- Early Detection and Prevention:
- Continuous monitoring of UFPs can facilitate the early detection of pollution spikes. By identifying periods and locations with high UFP concentrations, public health officials can issue timely warnings and take preventive measures to reduce exposure.
- Policy and Regulation:
- Data from UFP monitoring can inform policymakers and regulatory bodies, helping them to develop more effective air quality standards and regulations specifically targeting UFPs. This is crucial as traditional PM2.5 metrics may not adequately capture the health risks posed by UFPs.
- Targeted Interventions:
- Accurate UFP data can help target interventions more effectively. For example, areas with consistently high UFP levels might benefit from traffic reduction strategies, enhanced industrial emission controls, or the introduction of green spaces to improve air quality.
- Public Awareness and Behavior Change:
- Public access to real-time UFP data can increase awareness of air quality issues and encourage behavior changes that reduce exposure. For example, people might choose to limit outdoor activities during periods of high UFP concentration or take personal protective measures.
- Research and Development:
- Continuous monitoring provides valuable data for scientific research, helping to further understand the health impacts of UFPs and the effectiveness of various mitigation strategies. This data can drive innovation in pollution control technologies and public health interventions.
Current Monitoring Techniques
Traditional air quality monitoring methods focus on larger particles like PM10 and PM2.5, often missing the finer details provided by UFP data. These conventional methods typically use gravimetric analysis, where air samples are collected on filters and weighed, or optical methods that estimate particle mass concentration based on light scattering. However, these techniques are not sensitive enough to detect ultrafine particles (UFPs), which are smaller than 0.1 microns and often present in high numbers but low mass.
The primary limitation of traditional monitoring methods is their inability to detect and quantify particles at the nanometer scale. PM10 and PM2.5 monitors are designed to capture larger particulate matter and may not provide accurate readings for UFPs due to their lower mass and different physical properties. This means that areas with significant UFP pollution might appear to have acceptable air quality when measured with conventional methods, leading to an underestimation of the health risks associated with UFP exposure (Hinds, 1999).
Advanced Technologies: Condensation Particle Counter (CPC)
Advanced technologies, such as Condensation Particle Counters (CPC), have been developed to fill this gap. The CPC is a highly sensitive device that can detect and count particles as small as a few nanometers. It works by saturating the air sample with butanol vapor, which condenses onto the UFPs, enlarging them to a detectable size. These enlarged particles are then counted optically as they pass through a laser beam.
Advantages of CPC Technology
- High Sensitivity and Accuracy:
- CPCs can detect particles down to a few nanometers in diameter, providing a much more detailed picture of the particulate composition of the air. This high sensitivity is crucial for accurately assessing the concentration of UFPs, which are often present in significant numbers but not captured by traditional methods.
- Real-Time Monitoring:
- CPCs offer real-time monitoring capabilities, allowing for immediate detection of changes in UFP levels. This is particularly important for identifying pollution events and taking prompt action to mitigate exposure. Real-time data can also be used for epidemiological studies to correlate UFP exposure with health outcomes.
Current Efforts and Standardization
Efforts such as the ACTRIS network and European technical specifications (EN 16976 and CEN/TS 17434) are paving the way for standardized UFP measurement techniques. These initiatives aim to improve the reliability and comparability of UFP data, which is crucial for effective public health interventions.
Ultrafine particles, despite their tiny size, have a profound impact on human health. Understanding and monitoring these particles is essential for mitigating their adverse effects. Our advanced UFP measurement technology provides the necessary tools to accurately assess air quality and protect public health. By incorporating UFP measurements into regular air monitoring, we can identify high-risk areas, implement timely interventions, and ultimately improve public health outcomes.
Learn more about how our innovative UFP measurement solution can help you monitor air quality more accurately and protect against the health risks posed by ultrafine particles. Contact us today for a demonstration and start making a difference in air quality management.
- Buonanno, G., Stabile, L., & Morawska, L. (2009). Particle emission factors during cooking activities. Atmospheric Environment, 43(20), 3235-3242.
- Calderón-Garcidueñas, L., Solt, A. C., Henríquez-Roldán, C., Torres-Jardón, R., Nuse, B., Herritt, L., Villarreal-Calderon, R., Osnaya, N., Stone, I., García, R., Brooks, D. M., González-Maciel, A., Reynoso-Robles, R., Pérez-Guillé, B., & Díaz, P. (2008). Long-term air pollution exposure is associated with neuroinflammation, an altered innate immune response, disruption of the blood-brain barrier, ultrafine particulate deposition, and accumulation of amyloid beta-42 and alpha-synuclein in children and young adults. Toxicologic Pathology, 36(2), 289-310.
- Geiser, M., & Kreyling, W. G. (2010). Deposition and biokinetics of inhaled nanoparticles. Particle and Fibre Toxicology, 7, 2.
- Harrison, R. M., Jones, A. M., & Lawrence, R. G. (2012). A pragmatic mass closure model for airborne particulate matter at roadside and urban background sites. Atmospheric Environment, 46, 194-205.
- Hinds, W. C. (1999). Aerosol technology: Properties, behavior, and measurement of airborne particles. John Wiley & Sons.
- Hong, G., Jee, Y. (2020). Special issue on ultrafine particles: where are they from and how do they affect us? Experimental & Molecular Medicine, 52, 309-310
- Vezzani, B, Carinci M., Previati M., Giacovazzi S., Della Sala M., Gafa R., Lanza G., Wieckowski M.R., Pinton P., Giorgi C. (2022). Epigenetic Regulation: A Link between inflammation and carcinogenesis. Cancers (Basel), 14(5), 1221
- Kittelson, D. B. (1998). Engines and nanoparticles: A review. Journal of Aerosol Science, 29(5-6), 575-588.
- Li, N., Sioutas, C., Cho, A., Schmitz, D., Misra, C., Sempf, J., Wang, M., Oberley, T., Froines, J., & Nel, A. (2003). Ultrafine particulate pollutants induce oxidative stress and mitochondrial damage. Environmental Health Perspectives, 111(4), 455-460.
- Oberdörster, G., Oberdörster, E., & Oberdörster, J. (2005). Nanotoxicology: An emerging discipline evolving from studies of ultrafine particles. Environmental Health Perspectives, 113(7), 823-839.
- Seinfeld, J. H., & Pandis, S. N. (2016). Atmospheric chemistry and physics: From air pollution to climate change. John Wiley & Sons.
- Sioutas, C., Delfino, R. J., & Singh, M. (2005). Exposure assessment for atmospheric ultrafine particles (UFPs) and implications in epidemiologic research. Environmental Health Perspectives, 113(8), 947-955.
- Wang, S. C., & Flagan, R. C. (1990). Scanning electrical mobility spectrometer. Aerosol Science and Technology, 13(2), 230-240.
- Weichenthal S., Bai, L., Hatzopoulou M., Ryswyk K., Kwong, J.C., Jerrett, M., Donkelaar, A., Martin, R.V., Burnett, R.T., Lu, H., Chen, H. (2017) Long-term exposure to ambient ultrafine particles and respiratory disease incidence in Toronto, Canada: a cohort study. Environmental Epidemiology, 16, 64